Precipitation Strengthening/Hardening is a heat treatment technique used to increase the yield strength of many metal alloys. Especially the malleable metals such as the alloys of aluminum, magnesium, nickel, titanium, and some steels and stainless steels. Precipitation hardening is also called age hardening or particle hardening. Let us discuss more details about What is Precipitation Strengthening / Hardening.
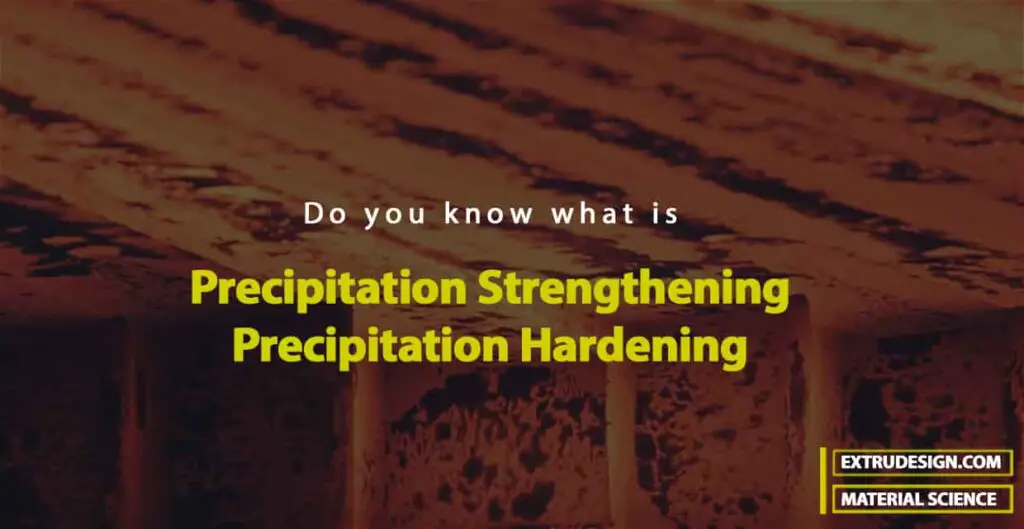
Precipitation Strengthening of a Generalized Binary Alloy
The object of precipitation strengthening is to create in a heat-treated alloy a dense and fine dispersion of precipitated particles in a matrix of deformable metal.
The precipitate particles act as obstacles to dislocation movement and thereby strengthen the heat-treated alloy. The precipitation-strengthening process can be explained in a general way by referring to the binary phase diagram of metals A and B shown below in figure 1.
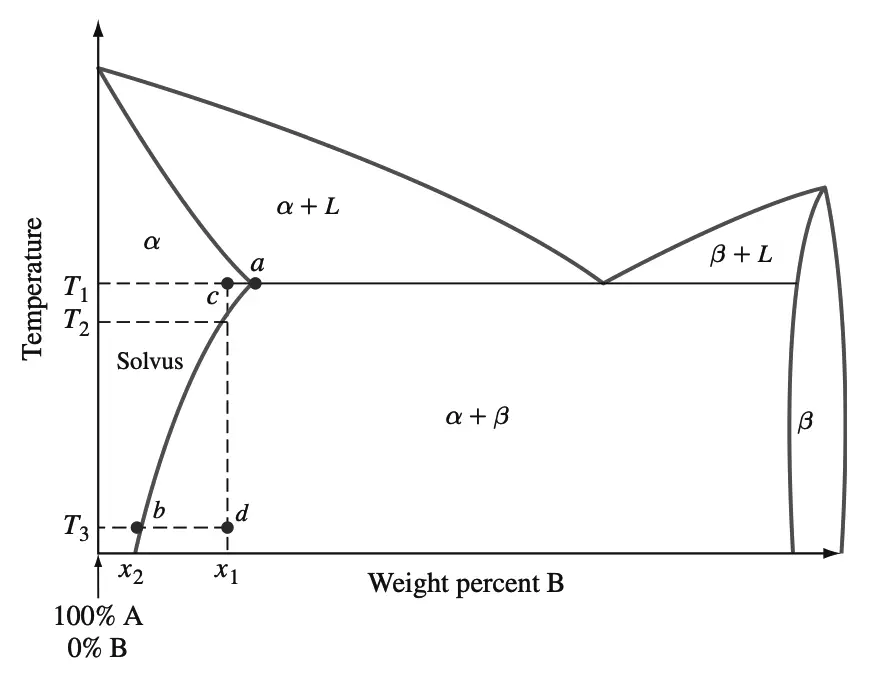
In order for an alloy system to be able to be precipitation-strengthened for certain alloy compositions, there must be a terminal solid solution that has a decreasing solid solubility as the temperature decreases.
The above phase diagram shows this type of decrease in solid solubility in terminal solid solution α in going from point a to point b along the indicated solvus.
Let us now consider the precipitation strengthening of an alloy of composition x1 of the above phase diagram. We choose the alloy composition x1 since there is a large decrease in the solid solubility of solid solution α in decreasing the temperature from T2 to T3.
The precipitation-strengthening process involves the following three basic steps:
- Solution heat treatment is the first step in the precipitation-strengthening process. Sometimes this treatment is referred to as solutionizing. The alloy sample, which may be in the wrought or cast form, is heated to a temperature between the solvus and solidus temperatures and soaked there until a uniform solid-solution structure is produced. Temperature T1 at point c of Figure 9.40 is selected for our alloy x because it lies midway between the solvus and solidus phase boundaries of solid solution α.
- Quenching is the second step in the precipitation-strengthening process. The sample is rapidly cooled to a lower temperature, usually room temperature, and the cooling medium is usually water at room temperature. The structure of the alloy sample after water quenching consists of a supersaturated solid solution. The structure of our alloy x1 after quenching to temperature T3 at point d of above phase diagram thus consists of a supersaturated solid solution of the α phase.
- Aging is the third basic step in the precipitation-strengthening process. Aging the solution heat-treated and quenched alloy sample is necessary so that a finely dispersed precipitate forms. The formation of a finely dispersed precipitate in the alloy is the objective of the precipitation-strengthening process. The fine precipitate in the alloy impedes dislocation movement during deformation by forcing the dislocations to either cut through the precipitated particles or go around them. By restricting dislocation movement during deformation, the alloy is strengthened.
Aging the alloy at room temperature is called natural aging, whereas aging at elevated temperatures is called artificial aging.
Most alloys require artificial aging, and the aging temperature is usually between about 15% and 25% of the temperature difference between room temperature and the solution heat-treatment temperature.
Decomposition Products Created by the Aging of the Supersaturated Solid Solution
A precipitation-hardenable alloy in the supersaturated solid solution condition is in a high-energy state, as indicated schematically by energy level 4 as shown in the below figure.
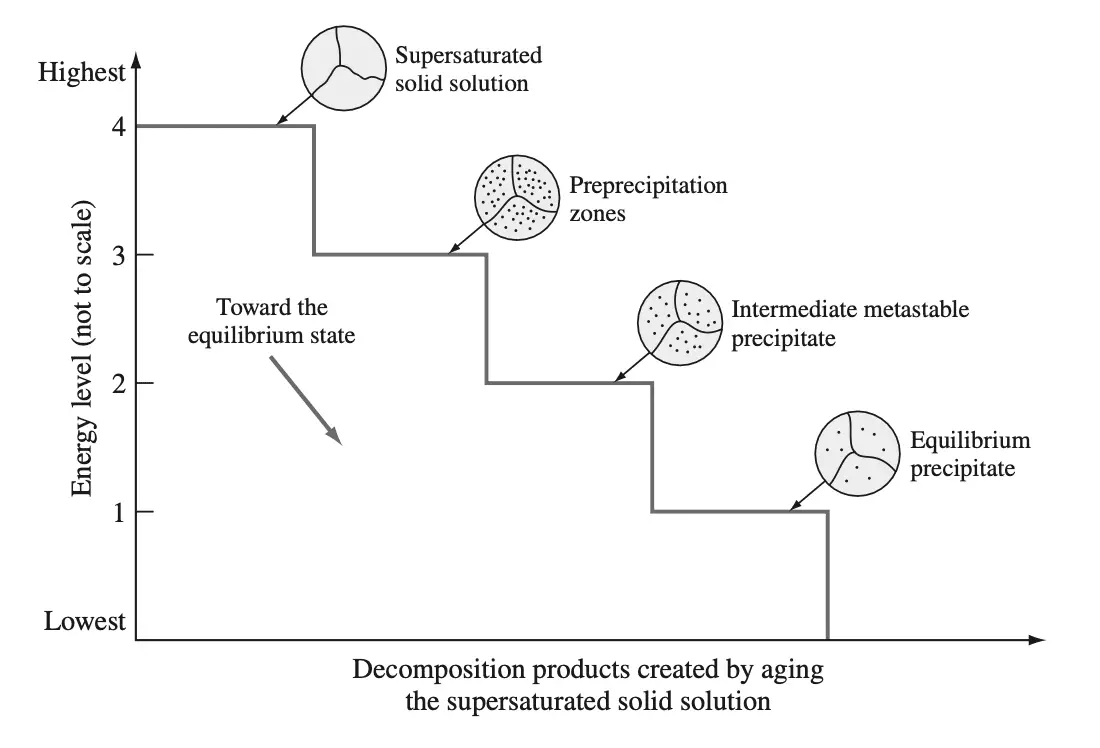
- This energy state is relatively unstable, and the alloy tends to seek a lower energy state by the spontaneous decomposition of the supersaturated solid solution into metastable phases or the equilibrium phases.
- The driving force for the precipitation of metastable phases or the equilibrium phase is the lowering of the energy of the system when these phases form.
- When the supersaturated solid solution of the precipitation-hardenable alloy is aged at a relatively low temperature where only a small amount of activation energy is available, clusters of segregated atoms called precipitation zones or GP zones, are formed.
- For the case of our alloy A-B of the above phase diagram (Figure 1), the zones will be regions enriched with B atoms in a matrix primarily of A atoms.
- The formation of these zones in the supersaturated solid solution is indicated by the circular sketch at the lower energy level 3 of Figure 2.
- Upon further aging and if sufficient activation energy is available (as a result of the aging temperature being high enough), the zones develop into or are replaced by a coarser (larger in size) intermediate metastable precipitate, indicated by the circular sketch at the still-lower energy level 2.
- Finally, if aging is continued (usually a higher temperature is necessary) and if sufficient activation energy is available, the intermediate precipitate is replaced by the equilibrium precipitate indicated by the even-still-lower energy level 1 of Figure 2.
The Effect of Aging Time on the Strength and Hardness of a Precipitation Hardenable Alloy that Has Been Solution Heat-Treated and Quenched
The effect of aging on strengthening a precipitation-hardenable alloy that has been solution heat-treated and quenched is usually presented as an aging curve. The aging curve is a plot of strength or hardness versus aging time (usually on a logarithmic scale) at a particular temperature. Below Figure 3 shows a schematic aging curve.
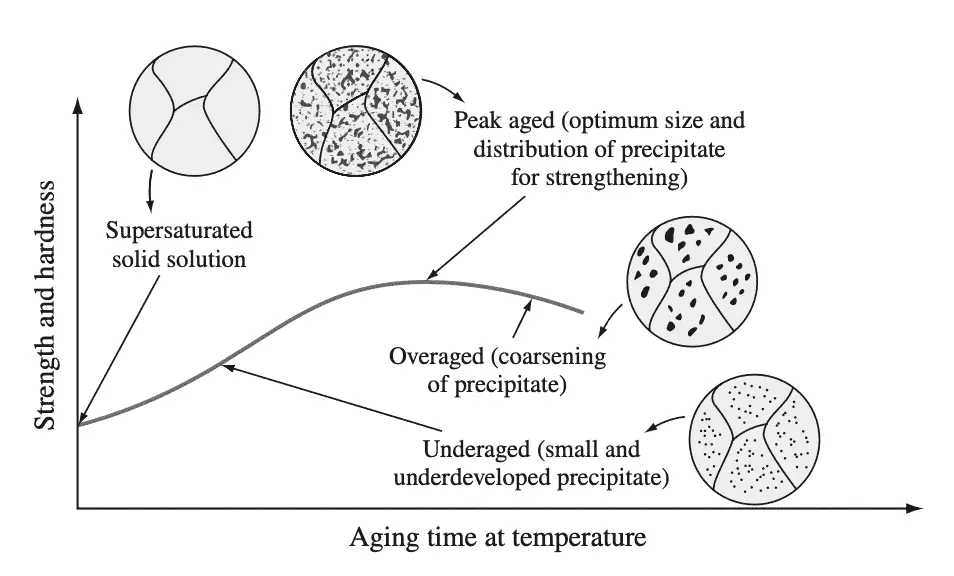
At zero time, the strength of the supersaturated solid solution is indicated on the ordinate axis of the plot. As the aging time increases, precipitation zones form, their size increases, and the alloy become stronger and harder and less ductile (Fig. 3).
A maximum strength (peak aged condition) is eventually reached if the aging temperature is sufficiently high, which is usually associated with the formation of an intermediate metastable precipitate. If aging is continued so that the intermediate precipitate coalesces and coarsens, the alloy overages and becomes weaker than in the peak aged condition (Fig. 3).
Precipitation Strengthening (Hardening) of an Al–4% Cu Alloy
examine the structure and hardness changes that occur during the precipitation heat treatment of aluminum–4% copper alloy.
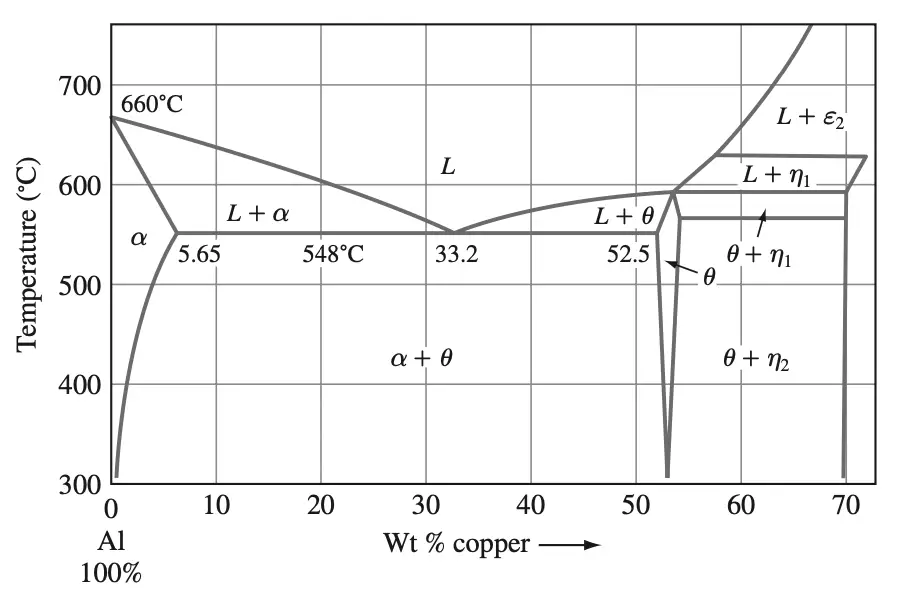
The heat-treatment sequence for the precipitation strengthening of this alloy is:
- Solution heat treatment: The Al–4% Cu alloy is solutionized at about 515°C (see the Al–Cu phase diagram of Figure 4).
- Quenching: The solution heat-treated alloy is rapidly cooled in water at room temperature.
- Aging: The alloy after solution heat treatment and quenching is artificially aged in the 130°C to 190°C range.
Structures Formed During the Aging of the Al–4% Cu Alloy
In the precipitation strengthening of Al–4% Cu alloys, five sequential structures can be identified:
(1) supersaturated solid-solution α,
(2) GP1 zones,
(3) GP2 zones (also called θ″ phase),
(4) θ′ phase, and
(5) θ phase, CuAl2.
Not all these phases can be produced at all aging temperatures. GP1 and GP2 zones are produced at lower aging temperatures and θ′ and θ phases occur at higher temperatures.
GP1 zones: These precipitation zones are formed at lower aging temperatures and are created by copper atoms segregating in the supersaturated solid-solution α. GP1 zones consist of segregated regions in the shape of disks a few atoms thick (0.4 to 0.6 nm) and about 8 to 10 nm in diameter, and they form on the {100} cubic planes of the matrix. Since the copper atoms have a diameter of about
11% less than the aluminum ones, the matrix lattice around the zones is strained tetragonally. GP1 zones are said to be coherent with the matrix lattice since the copper atoms just replace aluminum atoms in the lattice below figure 5. GP1 zones are detected under the electron microscope by the strain fields they create.
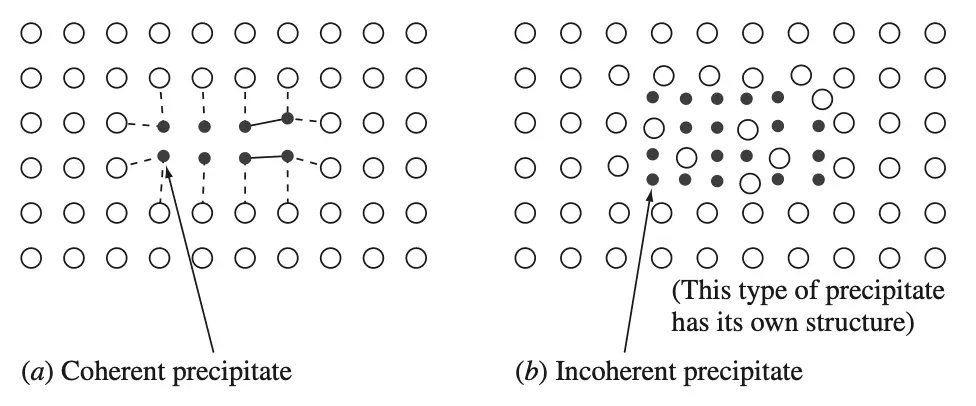
(a) a coherent precipitate
(b) an incoherent precipitate.
The coherent precipitate is associated
GP2 zones (θ″ phase): These zones also have a tetragonal structure and are coherent with the {100} of the matrix of the Al–4% Cu alloy. Their size ranges from about 1 to 4 nm thick to 10 to 100 nm in diameter as aging proceeds. θ′ phase. This phase nucleates heterogeneously, especially on dislocations, and is incoherent with the matrix. (An incoherent precipitate is one in which the precipitated particle has a distinct crystal structure different from the matrix [Figure 5a]). θ′ phase has a tetragonal structure with a thickness of 10 to 150 nm. θ phase. The equilibrium phase θ is incoherent and has the composition CuAl2. This phase has a BCT structure (a = 0.607 nm and c = 0.487 nm) and forms from θ′ or directly from the matrix.
The general sequence of precipitation in binary aluminum-copper alloys can be represented by
Supersaturated solid solution → GP1 zones → GP2 zones (θ″ phase) → θ′→ θ (CuAl2)
Correlation of Structures and Hardness in an Al–4% Cu Alloy
The hardness versus aging time curves for an Al–4% Cu alloy aged at 130°C and 190°C are shown in
below figure 6. At 130°C, GP1 zones are formed and increase the hardness of the alloy by impeding dislocation movement. Further aging at 130°C creates GP2 zones that increase the hardness still more by making dislocation movement still more difficult.
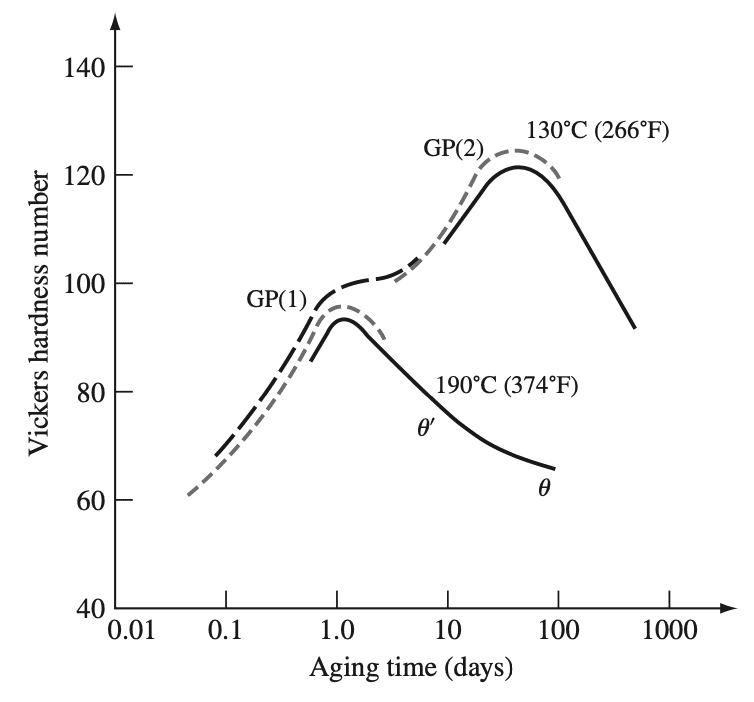
A maximum in hardness is reached with still more aging time at 130°C as θ′ forms. Aging beyond the hardness peak dissolves the GP2 zones and coarsens the θ′ phase, causing a decrease in the hardness of the alloy. GP1 zones do not form during aging at 190°C in the Al–4% Cu alloy since this temperature is above the GP1 solvus. With long aging times at 190°C, the equilibrium θ phase forms.
Let us solve an example problem to calculate the weight percentage.
Example problem to calculate weight percent for Aluminum alloy
Calculate the theoretical weight percent of the θ phase that could be formed at 27°C (room temperature) when a sample of Al–4.50 wt% Cu alloy is very slowly cooled from 548°C. Assume the solid solubility of Cu in Al at 27°C is 0.02 wt% and that the θ phase contains 54.0 wt% Cu.
Answer:
First, we draw a tie line xy on the Al–Cu phase diagram at 27°C between the α and θ phases, as shown in Figure 7a.
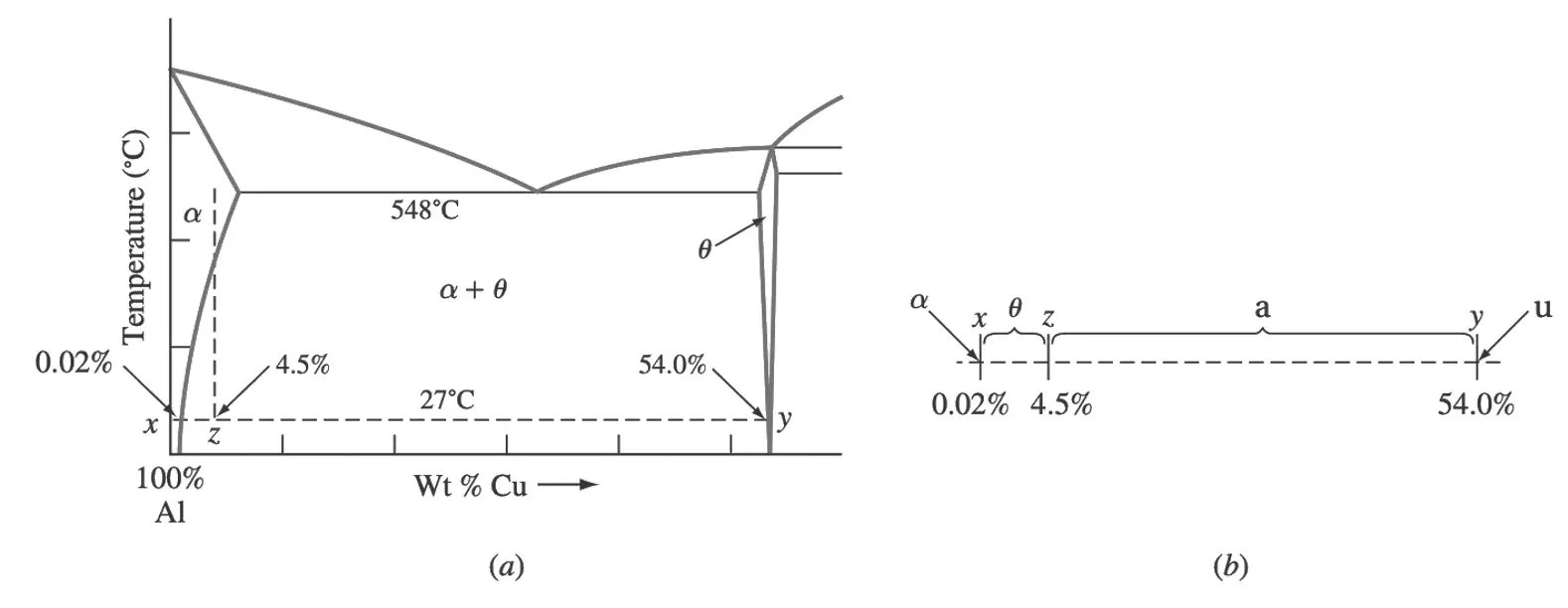
Figure 7b Isolated tie line xy indicating segment xz as representing the weight fraction of the θ phase.
Next, we indicate the 4.5% Cu composition point at z.
The ratio xz divided by the whole tie line xy as shown above figure 7b gives the weight fraction of the θ phase.
Thus,
θ wt% = [(4.50− 0.02) / (54.0 − 0.02)] x (100%)
θ wt% = 4.48 / 53.98 (100%)
θ wt% = 8.3%
Leave a Reply